What is meant by electronic transitions and spectroscopy?
The term “electronic” connotes electron, and the term “transition” implies transformation. In a molecule, the electrons move from a lower to a higher energy state due to excitation. The two energy states, the ground state and the excited state are the lowest and the highest energy states, respectively. An energy change is observed with this transition, which depicts the various data related to the molecule.
Spectroscopy involves the investigation of electromagnetic radiation comprising a specific wavelength. It helps in the quantitative and qualitative determination of the structure of the matter. All areas of science and technology utilize this analytical approach.
What are the types of electronic transitions?
The three kinds of electrons responsible for electronic transitions are:
- Sigma (σ) electrons in saturated molecules
- Pi (π) electrons in unsaturated molecules
- Nonbonding (n) electrons in nonbonded elements
These electrons absorb ultraviolet radiation, which causes excitation. The movement from the ground state to a higher energy state is categorized into four types of electronic transitions. They are as follows:
σ→σ* transition
In this transition, the electrons in a molecule move from a bonding (σ) orbital to its comparable anti-bonding (σ*) orbital. This transition takes place due to the electromagnetic radiation that gets absorbed. The highest quantity of energy is needed to undergo this transition. It can be observed in the methane molecule due to the presence of only C-H bonds.
n→σ* transition
In this transition, the electrons from a nonbonding orbital (n) move to an anti-bonding (σ*) orbital. The lowest quantity of energy is needed to undergo this transition. Halogens and elements like sulfur, oxygen, and nitrogen display this transition.
n→π* transition
In this transition, the electrons from a non-bonding orbital (n) move to an anti-bonding (π*) orbital. The lowest quantity of energy is needed to undergo this transition. Halogens and elements like sulfur, oxygen, or nitrogen display this transition.
π→π* transition
In this transition, the electrons move from a bonding orbital (π) to an anti-bonding (π*). Its energy requirement ranges in between the energies required for (n→π*) and (n→σ*). Organic compounds like the aromatic ones, alkenes, alkynes, nitriles, and carbonyl compounds display this transition.
Some transitions between energy levels are radiative, and some are nonradiative. The photon absorption involved between two energy levels is a radiative transition, whereas a transition involving no photons between two energy levels is a nonradiative transition.
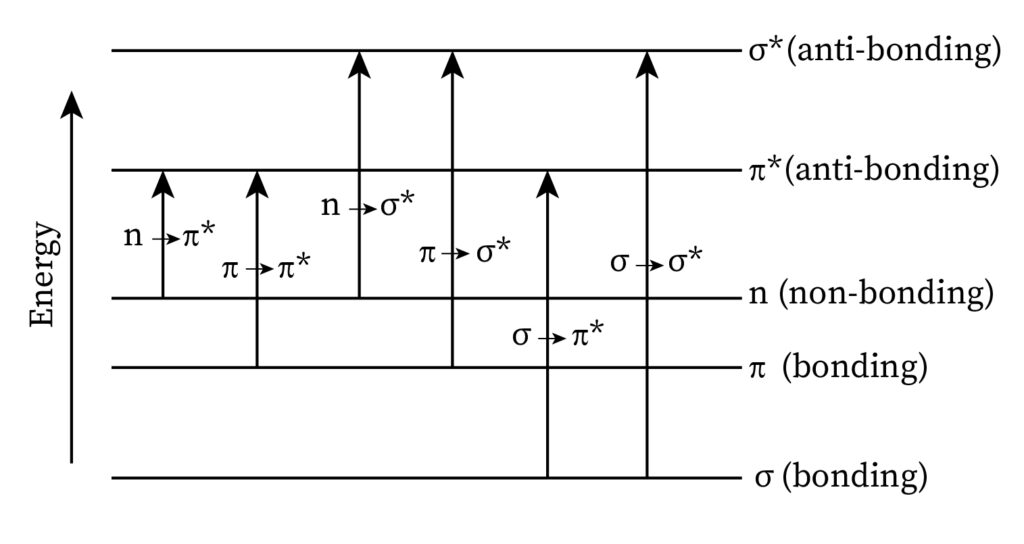
Spectroscopy
Spectroscopy focuses on examining the interaction occurring between electromagnetic radiation and matter, where the radiation’s wavelength operates as a function. Its advancement has paved the way for analyzing the interaction occurring in different particles through dependence on collision energy.
A particular beam of electromagnetic radiation is made to interact with a given sample. The electrons absorb the electromagnetic radiation, and they get excited. They move to the excited state from the ground state and further get de-excited when they return to their ground state by emitting light radiations.
Spectroscopy provides information about the structural aspect of atoms or molecules. It discovers the composition and quantity of a given sample, which is possible due to the distant reflection depicted by each atom or molecule. A graph of the intensity of absorbed/emitted light versus frequency or wavelength is plotted to obtain the spectrum, and then this spectrum is measured by the spectrometer.
Beer Lambert’s law
Beer Lambert’s law is the principle on which an instrument named a spectrophotometer operates. A source emits light of different intensities, which passes through the monochromator that converts distinct colors into a single color. The light is incident on the cuvette containing the sample, and it gets transmitted further. A detector is placed which measures the light intensity and converts it into an electrical signal.
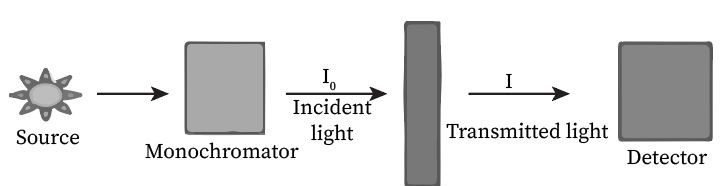
The measurement of absorbed light increases with an increase in the concentration of the sample, according to Beer’s law. However, it also increases with path length, which is stated by Lambert’s law. The combination of both gives rise to Beer Lambert’s law, and its equation is as follows:
Here,
A = Absorbance
ε= Molar absorption coefficient
c = Molar concentration of the sample
l = optical path length
Io = Intensity of incident light
I = Intensity of emitted light
Types of spectroscopy
Since spectroscopy deals with the estimation of interaction between electromagnetic radiation and samples; hence, either absorption, emission, or the scattering process is observed, classifying spectroscopy into three types.
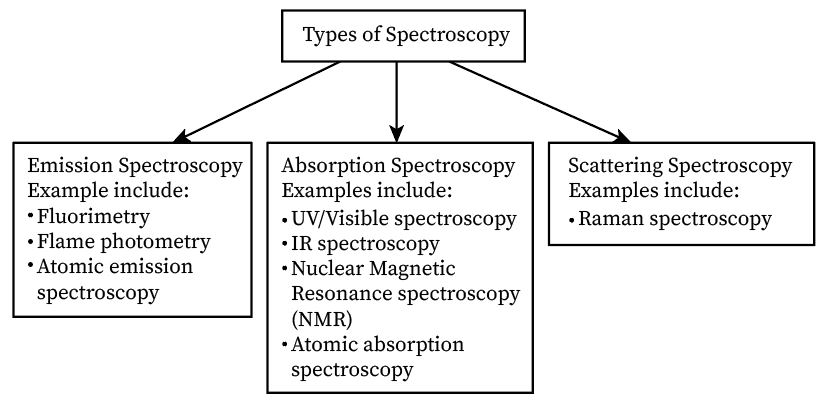
Absorption spectroscopy
Absorption spectroscopy is a spectroscopic method of absorbing electromagnetic radiation when the sample and the radiation display interaction. The wavelength or frequency is used as a function. The spectrum obtained from absorption is called an absorption spectrum.
The examples of absorption spectroscopy are as follows:
- Atomic Absorption (AA) Spectroscopy.
- Ultraviolet (UV)/ Visible Spectroscopy.
- Infrared (IR) Spectroscopy.
- Nuclear Magnetic Resonance (NMR) spectroscopy.
Emission spectroscopy
Emission spectroscopy is a spectroscopic method in which atoms/molecules emit photons while undergoing an electronic transition, that is, from a lower to a higher energy state. The photon’s wavelength can be captured and examined to discover the sample’s composition. The emission and absorption spectra differ from one another as the emission spectra consist of colored lines while the absorption spectra consist of dark lines.
The examples of emission spectroscopy are as follows:
- Atomic Emission (AE) spectroscopy.
- Flame photometry.
- Fluorimetry.
Scattering spectroscopy
Scattering spectroscopy is a spectroscopic method by which a molecule’s vibrational and rotational states are attained when light scattering occurs, leading to the excitement of the atoms. It rectifies the groups in the sample, which further helps to detect its composition. This process is the fastest compared to absorption and emission processes.
The example of scattering spectroscopy is as follows:
- Raman Spectroscopy.
Common Mistakes
The common mistakes encountered while using spectroscopic methods are the neglection of material selection of cuvette, based on the spectroscopic method used, solvent selection, and calibration of instruments.
- In ultraviolet visible spectroscopy, the sample should be placed in a quartz or silica glass cuvette because they are transparent, causing no interference and cannot absorb the UV energy.
- The solvent selection should be done carefully so that it does not get absorbed in the given region. Solvent directly alters the spectrum’s shape and quality.
- Calibration should be performed to ensure the accuracy of the instrument, and this is done by placing a blank cuvette inside the instrument.
Context and Applications
This topic is significant in the professional exams for both undergraduate and postgraduate courses, especially for:
Bachelors in Chemistry
Masters in Material Science
Masters in Pure and Applied Chemistry
Masters in Analytical Chemistry
Bachelors in Biochemistry
Masters in Biochemistry
Related Concepts
Spectroscopic Methods
Atomic Structure
Electromagnetic Spectrum
Analysis of Compounds
Practice Problems
Q1: Which type of electronic transition do aromatic compounds undergo?
(a) (σ→σ*)
(b) (n→σ*)
(c) (n→π*)
(d) (π→π*)
Correct option: (d)
Q2: On which of the following factors does absorbance depend?
(a) Molar concentration of the sample
(b) Path length
(c) Molar absorption coefficient
(d) All of the above
Correct option: (d)
Q3: Nuclear Magnetic Resonance (NMR) spectroscopy is an example of which type of spectroscopy?
(a) Absorption spectroscopy
(b) Emission spectroscopy
(c) Scattering spectroscopy
(d) None of the above
Correct option: (a)
Q4: Which type of spectra display colored lines?
(a) Absorption spectra
(b) Emission spectra
(c) Scattering spectra
(d) All of the above
Correct option: (b)
Q5: What is the unit of absorbance?
(a) Liters per mole per cm (L/mol.cm)
(b) Moles per liter (mol/L)
(c) Unitless
(d) Centimeters (cm)
Correct option: (c)
Want more help with your chemistry homework?
*Response times may vary by subject and question complexity. Median response time is 34 minutes for paid subscribers and may be longer for promotional offers.
Electronic Transitions and Spectroscopy Homework Questions from Fellow Students
Browse our recently answered Electronic Transitions and Spectroscopy homework questions.