Significance The optix gene is well known for its genetic association with wing pattern variation in butterflies; however, its actual function has never been directly confirmed. Using CRISPR genome editing in multiple butterfly species, we show that this gene plays a fundamental and deeply conserved role in the butterfly family Nymphalidae, where it acts as an activator of wing color. We were also surprised to discover that optix simultaneously controls blue iridescence in some species as well, providing an example of how a single gene can act as a switch to coordinate between structural and pigmentary coloration. Abstract also show that optix simultaneously acts as a switch gene The optic gene has been implicated in butterfly wing pattern adaptation by genetic association, mapping, and expression studies. The actual developmental function of this gene has remained unclear, however. Here we used CRISPR/Cas9 genome editing to show that optix plays a fundamental role in nymphalid butterfly wing pattern development, where it is it is required for determination of all chromatic coloration opti knockouts in four species show of show complete replacement of color pigments with melanins, with corresponding changes in pigment-related gene expression, resulting in g in black and gray butterflies. We structural iridescence in some butterflies, demonstrating simple regulatory coordination of structural and pigmentary of coloration. Remarkably, these optix knockouts phenocopy the recurring "black and blue" wing pattern archetype that has arisen on many independent occasions in butterflies. Here we demonstrate a simple genetic basis for structural coloration, and show that optix plays a deeply conserved role in butterfly wing patter development. Sign up for PNAS alerts. Get alerts for new articles, or get an alert when an article is cited Butterfly wing patterns provide an important model system for studying the interplay among ecological developmental and genetic factors in the evolution of complex morphological traits. Dozens of genes have been implicated in wing pattem development thanks to a combination of comparative expression and, more recently, knockout studies (1-4). Interestingly, however, mapping and association work has highlighted only a small subset of these genes that seem to play a all been genetically a y associated with local adaptation in multiple Pedicure gook A, care and doublesex (5-3). These genes are nadas "adaptive hotspor genes chic logical and/or r acrosse d. based on detailed crossing and expression studies. these genes behave as complex trait regulators, with different alleles associated with different spatial expression doners that determine highly varied and complex color patterns, not simply the presence or absence of individual features. Although there is strong interest in these genes for these reasons, their specific developmental roles and the depth of conservation of their color patteming functions remain unclear. Here we present a comparative functional analysis of the optix gene in buterflies. This gene is linked to adaptive geographic variation of red ommochrome color pattems in the genus Heliconius, although its actual function remained unconfirmed before the present study (5, 13), optic is al is also interesting because it is expressed in association with nonpigmentation wing traits in various species, including morphologically derived wing conjugation scales, suggesting that it may have multiple regulatory roles in both wing scale coloration and structure (5, 14), in the present work, we used Case-mediated targeted deletion of optic to test its color pattering function in four species of nymphalid butterflies. Not only did we confirm deeply conserved roles for optix i surveyed, but we were surprised to find that in chology in all species al iridescence in some butterflies. Importantly, this coordinated regulation of pigmentation and indescence strongly phenocopies wing pattems seen in other distantly related species, leading us to hypothesize that optix may have played a role in wing pattern evolution in many different butterfly lineages. Results optix Simultaneously Represses Melanins and Promotes Ommochromes. optix was first identified as a wing pattern gene candidate in Heliconius butterflies, in which mapping, association, and in situ expression data suggest a role in the determination of red color patterns (5, 14, 15). Subsequent mRNA-seq work also showed up-regulation of optix in red color patterns of the painted lady butery Vanessa card, raising the possibility of a more widespread role for this gene in red color pattern specification (16). To functionally confirm the role of optix in color patterning, we used a Cas-mediated long-deletion mosaic knockout approach (4, 16, 17) in four nymphalid species: Heliconius erat, Agraulis variae, V. cardul, and Junonia conia (Dataset S1, Tables S1 and S2). optix knockout in H. erato produced results predicted by previous genetic and in situ hybridization studies. Mosaics revealed loss of the red color patiems previously shown to be presaged by pupal optix expression, including the color feld at the base of the forewing (the so-called 'dennis" element) and the hindwing rays (Fig. 1A and B and Dataset S1, Tables S1 and S2). Not only was red pigmentation lest in knockout clones, but red pigments were replaced by black pigments. These results show that optix is required for red color pattern specification in H. erato, and acts as a coordinating "or" switch between ormochrome (orange and red) and melanin (black and gray) pigment types. Fig. 1. optix determines wing scale color identity and morphology in H. erato and A. vanilee. (A) optix mosaic knockouts in H erato result in conversion of red ommochrome color patiems to black melanin. The comparisons shown are left-right asymmetrical knockout effects from single individual injected buterflies. (B) Detail of mutant clone highlighted in the mutant in A showing red replaced by black in a proximal red "dennis" pattern of the dorsal forewing. (C-C) optix knockout mosaics showing transformation of pointed wing conjugation scales to normal wing scales. Each panel in the series shows successive detail. (D) opdix replaces orange and brown ommochromes in A. vanillae with melonins, resulting in a black and silver butterfly. Arrows highlight presumptive clone boundaries discussed in the text. () Detail of a knockout done boundary highlighting the switch between red and black pigmentation in the ventral forewing from D. (F) Ventral view of black spots in optix knockout mutant showing a phenotype similar to WT. (G and G) Wing conjugation scales in WT (G) and optix knockout mutant (G) demonstrating a role for optic in determining A. vanilla scale morphology To test whether optix has a role in color paterning in a more basal heliconine butterfly, we generated knockouts in the gulf fritillary A. vanilla (Fig. 1D-F, Fig. S1, and Dataset 51, Tables S1 and S2). Previous in situ hybridization work in A vanilla suggested that optic is not expressed in association with ommochrome patems during early pupal development. leading to the hypothesis that the gene might not play a major color patterning role in this species (14). Thus, we were surprised to find that optix knockout resulted in a complete transformation of ommochrome scales to black melanin scales, producing a very unusual and dramatic phenotype of a completely black and silver butterfly (Fig. 10). We also observed a handful of orange or brown scales that changed to silver patches (Fig. 10, ventral forewing. 1. green arrows), although we cannot confidently conclude that these are cell-autonomous knockout effects s 3 since it has been shown that silver scales can be induced through long-range signaling (18, 19), in this case potentially from neighboring knockout clones. The with type (WT) black spots and marginal bands in the ventral forewing were unaffected in knockouts and remained a darker color relative to the neighboring mutant melanic scales (Fig. 1), optix knockout also resulted in melanic hyperpigmentation in adult bodies (Fig. S14). Thus, our results in A. vanillae are consistent with those in Herato in supporting a role for optix as a switch-like regulator that toggles between ormochrome and melanin pattems. Fig. S1 A. vanile WT and optic knockout phenotypes in developing wings and body. Ilustrative optix mosaic knockout phenotypes are shown at different stages of development (A) adult, (B) ommochrome stage (when ommochrome pigments first appear). (C) melanin stage (when black melanin pigments first began to appear), and (D) late melanin stage (when black melanin pigments are prevalent across the wing). Arrowheads and dashed lines highlight ilustrative mutant done boundaries. We next aimed to test whether optix regulates wing patterning in more distantly related lineages by performing knockouts in the nymphalines V. cardul (Fig. 2, Fig. 52, and Dataset S1, Tables S1 and S2) and J. conia (Fig. 3, Fig. 53, and Dacase S1, Tables S1 and S2), which diverged from heliconines by 75-80 mya (20, 21). Our results were consistent with those from H. erato and A. vanillae, where optir knockouts in both species showed mutant clones with complete loss of presumptive ommochrome pigments and replacement by melanins (Figs. 2A-E and 3A-C). One interesting exception to this finding was in V. cardul, where the complete ommochrome-to-melanin switch consistently occurred in dorsal wings (Fig. 2A and B), but much of the ventral wing area showed only a loss of ommochrome and little obvious hypermetanization (Fig. 2A, C, and D). Importantly, however, we recovered late-stage pupal wings from V. card that had died before emergence that displayed hypermnelanization of ventral wing surfaces (Fig. 3E). We speculate that this variable strength of ventral wing pattern melanization among individuals may reflect a dosage effect, with the stronger phenotypes representing biallelic optix deletion clones. We have no direct evidence for this, however, given the challenges in rigorously characterizing specific alleles from individual mutant clones (1). We also recovered hypermanic opti knockout pupae in both V. cardul (Fig. S2) and J. coenia (Fig. S3). Taken together, our knockout data from four nymphalids clearly demonstrate that optic plays a conserved role in coordinating the color identities of butterfly wing scales, s, where it operates as an "or" function between ommochrome and melanin identities, but a ut also may be modulated to serve as an "and" function in some contexts, as demonstrated by phenotypes seen in the ventral wings of V. cardu Fig. 2. optic determines wing scale color identity and morphology in V. cardul. (A) optix knockout mutant showing loss of omnochrome pigments (8-0) Left-right asymmetrical comparisons from individual optix mutant butterflies, showing melanization of red pattems (8), loss of color pigmentation without widespread hypermelanization in the ventral forewing (C) and hindwing (D). (E) Severe defects in late-stage pupal wings displaying hypermelanization in sed regions of dorsal and ventral wing surfaces (green and purple arrowheads) compared with mosaic adult mutants in A. (F) opdx knockout showing conversion of pointed wing conjugation scales to normal scales. Fig. 3. optic coordinates pigment color and structural iridescence in J. conia. (A) optix knockout results in loss of red onochrome pigments, r s melanization, and gain of structural iridescence. The left-right comparisons shown are bilaterally asymmetric mosaic phenotypes from single individuals. Wings to the right are additional examples of mosaic mutants with clearly identifiable knockout clones that highlight indescence in both dorsal and ventral wing surfaces. (B-C) Details of mosaic defects in the asymetic mutant shown in A, including strong induced indescence in c in dorsal discal spot (D) and eyespot ring (8), replacement of orange ommochrome with presumptive melanin in ventral discal spot (DI) red patiens (C), and detail of knockout-induced indescence on the ventral hindwing (D). (E) Mosaic knockout clones showing asymmetrical variation in promoted melanin and iridescence induction in dorsal forewings. (F) Wing conjugation scales in WT and optir knockout mutant Fig. 52. V. card WT and optix knockout phenotypes in developing wings and pupae. Mustrative optimes knockout phenotypes are shown at different stages of development (A) adult and pupa. (B) ommochrome stage (when omnochrome pigments first appear). (C) melanin stage (when black melanin pigments first began to appear), and (D) melanin stage (when black melanin pigments are prevalent across the wing). Arrowheads and dashed lines highlight lustrative mutant clone boundaries. The actual wings used for the RNA-seq experiments shown in Fig. 5 are labeled Fig. 52 J.conia WT and optix knockout phenotypes in developing wings and pupae. Mustrative optix mosaic knockout phenotypes are shown at different stages of development (4) whole adults and pupa, (0) details of clone boundaries on adult wings showing iridescent and noniridescent color shifts, and (C) late melanin stage wings (when black melanin pigments are prevalent across the wing). Arrowheads and dashed lines highlight ilustrative mutant clones and boundaries. The actual wings used for RNA-seq experiments in Fig. 5 are labeled "RNA-seq" optix Function Is Required for Determination of Derived Scale Structures. Along with its expression in color patios, in situ optix expression also precisely predicts the location of patches of derived, pointed scales thought to play a role in conjugating forewings and hindwings during fight (5,14). To determine whether optix plays a role in determining the unusual morphology of these scales, we examined optix knockouts for changes in wing scale structure. Indeed, we found that in all four species, optic knockout resulted in transformation of One 1 vanillae (14) scales occurred within obvious knockout clones. Therefore, whether optic plays a functional role in the development of es in transformation of wing conjugation scales to role in switching between discrete complex scale morphologies in buying optix Regulates Iridescence in J. coena The most surprising results from the present study came from our work in 2. conia, where knockout of op induced strong blue indescence in wing scales (Fig. 3A-E and Fig. 5 This induction of structural color occurred in addition to the loss of home pigmentation described above Broad strong descence occumed in knockdal wing surfaces, including in scales that are normally buff or orange in WT bueres, such as the big orange discal spots and eyespot rings (Fig. 3A and d) and the marginal bands of the dorsal hindeling (Fig. 34) descence induction was areas of the wing that are normally heavily melanced in WT indus, such as the black borders of the dial spets regulatory effect. We speculate that these dunes may represent lower dosage effects due to clones being moncadelic for deletion; however, we have not confirmed this hypothesis in sum, our knockout experiments in Jona addition to its other function pigmentation J. ceenia optix Mutants Phenocopy Distantly Related Species. One striking aspect of the J. conie pockout phenotype is the degree to which peoples the arch" tailles. Even singly Rocusing on the nynghald vibe Junonin which includes J. coena phylogenetic analysis suggests Fig 4 Notable examples of fed black and blue phenotypes are seen in such species as Junonia at which are knockouts. As in V card wings and are artholings or panings of putate pigeration genes exqu pignant maturation andor spatially associated with sed and black color sgions in card Using these criteria w ochrome pigmentation and 3 genes associated with melanin pigmentation V porters and one ATP-binding capo fany meber Another strongly down-regulated Juvenile hormone binding pro a gene of unknown function that showed one of the strangest sig expression levels with log-ble change in Pyke FDR yakaca and J. conia ined by local changes in optix expression, although further work is needed to test this hypothes pem density seen in bus candu and showed ively low on hagments per base of transorgt per million mapped pigment pers. Furthermore, our data suggest that there may be differences in the downs of the opt s that we investigated, optikas producing and replaced with black and gray means. Further bucke biochemical, and morphological features, has such a simple an dically alter aange of independent as Compared with her wing body. The behaves the a paidush that can be applied anywhere on a tutely to model and the can be deployed across numerous morphologies to effect disc phenotypic shits through switch- ow a gene underlying adaptive evolution also has a deeply conserved regulatory role in morphological development. In this respect it is investing that opt knockouts phonocopy the di red-black-descent evolutionary color state changes commonly observed in many wing patiem elements, including the whole wing black and blue parchetype that has an on any occasions in buteres. These phenols lead us to hypothesize that evolutionary changes in cox expression may have played a repeated rede in nymphalid wing pate evolution, although additional functional work is needed to rigorously as this hypothes Materials and Methods CRISPR/Cas Genome Editing design and unce Phylogenetic Analysis causing maximum parsimony in the character We first divided merly wings in four segions based border try, and discal spor so called D and D pam) We further classfied the descence vedic 0343234 and depending on the extent of descence occurence in these foun Pupal Wing Isolation and mRNA extraction cadandofwings and hidings were added and stored in The Tybalts were sandbewing and wi the optix knockout effects seen in conia (C) Parsimony reconstruction of indescence in Junoni suggests origins as a fixed phenotype. Species highlighted in red are shown in A. Prevalence of descence analing Global Expression Profiling of Butterfly Wings in Response to optix Knockout. To imate how wing patems are controlled by optix, we used RNA-seq to compare transcript abundance in WT and s are visible in two biological seplicates of both W and strong knockout phenotypes (Figs. 52 and 53 and Dataset 51, Table 5) West examined the expession of op and script ads taled to reveal any paid vansoripes showing lesions at the Cast out site, suggesting expressed genes (DEG) in comparisons between WT and optix knockout wings using cutoff values of a old change of and a false discovery case (FDR) of in card SP ungenes were up-regulated and 243 were dove-regulated inclved in Pane pathway and drophis eye development (1) S1 Table 57 highlights Discussion functional evidence that the widely studied a ot plays a fundamen The ability of a single gene to independ the placement of color anywhere only allo dass of pigments papilodones in papiliorids, prins in pledds, and comes in nymphalids (1) Given th dent origina in butterflies. We propose that opt kaly played a causative role in the origin of wing color in the Nymphalidae, the most speciose and morphologically diverse butterfly family. This gene's simple and deeply conserved regulatory function of replacing melanin with onochrome pigmentation leads to a simple model where co-option of optic into a color patting role could have been the key event sufficient for the deployment of we collected in J. conia Library construction and sequencing were conducted as described previously Analysis of Transcript Expression Data The card come by was downloaded from www.bugome.org and served buldance for conia A-seq analysis sequencing reads from this study and National Cer for Beech using Trinity in scenon The TansDecoder predicted geneset was then searched agai Pan and GO-debuses for gene, domain, and GO annotation, respectively Sequencing data were subrced to quality color and that has also played a nole in color parten evolution in mutiple species (25) The stage of ably and expression profes are able in the GED database accession
Significance The optix gene is well known for its genetic association with wing pattern variation in butterflies; however, its actual function has never been directly confirmed. Using CRISPR genome editing in multiple butterfly species, we show that this gene plays a fundamental and deeply conserved role in the butterfly family Nymphalidae, where it acts as an activator of wing color. We were also surprised to discover that optix simultaneously controls blue iridescence in some species as well, providing an example of how a single gene can act as a switch to coordinate between structural and pigmentary coloration. Abstract also show that optix simultaneously acts as a switch gene The optic gene has been implicated in butterfly wing pattern adaptation by genetic association, mapping, and expression studies. The actual developmental function of this gene has remained unclear, however. Here we used CRISPR/Cas9 genome editing to show that optix plays a fundamental role in nymphalid butterfly wing pattern development, where it is it is required for determination of all chromatic coloration opti knockouts in four species show of show complete replacement of color pigments with melanins, with corresponding changes in pigment-related gene expression, resulting in g in black and gray butterflies. We structural iridescence in some butterflies, demonstrating simple regulatory coordination of structural and pigmentary of coloration. Remarkably, these optix knockouts phenocopy the recurring "black and blue" wing pattern archetype that has arisen on many independent occasions in butterflies. Here we demonstrate a simple genetic basis for structural coloration, and show that optix plays a deeply conserved role in butterfly wing patter development. Sign up for PNAS alerts. Get alerts for new articles, or get an alert when an article is cited Butterfly wing patterns provide an important model system for studying the interplay among ecological developmental and genetic factors in the evolution of complex morphological traits. Dozens of genes have been implicated in wing pattem development thanks to a combination of comparative expression and, more recently, knockout studies (1-4). Interestingly, however, mapping and association work has highlighted only a small subset of these genes that seem to play a all been genetically a y associated with local adaptation in multiple Pedicure gook A, care and doublesex (5-3). These genes are nadas "adaptive hotspor genes chic logical and/or r acrosse d. based on detailed crossing and expression studies. these genes behave as complex trait regulators, with different alleles associated with different spatial expression doners that determine highly varied and complex color patterns, not simply the presence or absence of individual features. Although there is strong interest in these genes for these reasons, their specific developmental roles and the depth of conservation of their color patteming functions remain unclear. Here we present a comparative functional analysis of the optix gene in buterflies. This gene is linked to adaptive geographic variation of red ommochrome color pattems in the genus Heliconius, although its actual function remained unconfirmed before the present study (5, 13), optic is al is also interesting because it is expressed in association with nonpigmentation wing traits in various species, including morphologically derived wing conjugation scales, suggesting that it may have multiple regulatory roles in both wing scale coloration and structure (5, 14), in the present work, we used Case-mediated targeted deletion of optic to test its color pattering function in four species of nymphalid butterflies. Not only did we confirm deeply conserved roles for optix i surveyed, but we were surprised to find that in chology in all species al iridescence in some butterflies. Importantly, this coordinated regulation of pigmentation and indescence strongly phenocopies wing pattems seen in other distantly related species, leading us to hypothesize that optix may have played a role in wing pattern evolution in many different butterfly lineages. Results optix Simultaneously Represses Melanins and Promotes Ommochromes. optix was first identified as a wing pattern gene candidate in Heliconius butterflies, in which mapping, association, and in situ expression data suggest a role in the determination of red color patterns (5, 14, 15). Subsequent mRNA-seq work also showed up-regulation of optix in red color patterns of the painted lady butery Vanessa card, raising the possibility of a more widespread role for this gene in red color pattern specification (16). To functionally confirm the role of optix in color patterning, we used a Cas-mediated long-deletion mosaic knockout approach (4, 16, 17) in four nymphalid species: Heliconius erat, Agraulis variae, V. cardul, and Junonia conia (Dataset S1, Tables S1 and S2). optix knockout in H. erato produced results predicted by previous genetic and in situ hybridization studies. Mosaics revealed loss of the red color patiems previously shown to be presaged by pupal optix expression, including the color feld at the base of the forewing (the so-called 'dennis" element) and the hindwing rays (Fig. 1A and B and Dataset S1, Tables S1 and S2). Not only was red pigmentation lest in knockout clones, but red pigments were replaced by black pigments. These results show that optix is required for red color pattern specification in H. erato, and acts as a coordinating "or" switch between ormochrome (orange and red) and melanin (black and gray) pigment types. Fig. 1. optix determines wing scale color identity and morphology in H. erato and A. vanilee. (A) optix mosaic knockouts in H erato result in conversion of red ommochrome color patiems to black melanin. The comparisons shown are left-right asymmetrical knockout effects from single individual injected buterflies. (B) Detail of mutant clone highlighted in the mutant in A showing red replaced by black in a proximal red "dennis" pattern of the dorsal forewing. (C-C) optix knockout mosaics showing transformation of pointed wing conjugation scales to normal wing scales. Each panel in the series shows successive detail. (D) opdix replaces orange and brown ommochromes in A. vanillae with melonins, resulting in a black and silver butterfly. Arrows highlight presumptive clone boundaries discussed in the text. () Detail of a knockout done boundary highlighting the switch between red and black pigmentation in the ventral forewing from D. (F) Ventral view of black spots in optix knockout mutant showing a phenotype similar to WT. (G and G) Wing conjugation scales in WT (G) and optix knockout mutant (G) demonstrating a role for optic in determining A. vanilla scale morphology To test whether optix has a role in color paterning in a more basal heliconine butterfly, we generated knockouts in the gulf fritillary A. vanilla (Fig. 1D-F, Fig. S1, and Dataset 51, Tables S1 and S2). Previous in situ hybridization work in A vanilla suggested that optic is not expressed in association with ommochrome patems during early pupal development. leading to the hypothesis that the gene might not play a major color patterning role in this species (14). Thus, we were surprised to find that optix knockout resulted in a complete transformation of ommochrome scales to black melanin scales, producing a very unusual and dramatic phenotype of a completely black and silver butterfly (Fig. 10). We also observed a handful of orange or brown scales that changed to silver patches (Fig. 10, ventral forewing. 1. green arrows), although we cannot confidently conclude that these are cell-autonomous knockout effects s 3 since it has been shown that silver scales can be induced through long-range signaling (18, 19), in this case potentially from neighboring knockout clones. The with type (WT) black spots and marginal bands in the ventral forewing were unaffected in knockouts and remained a darker color relative to the neighboring mutant melanic scales (Fig. 1), optix knockout also resulted in melanic hyperpigmentation in adult bodies (Fig. S14). Thus, our results in A. vanillae are consistent with those in Herato in supporting a role for optix as a switch-like regulator that toggles between ormochrome and melanin pattems. Fig. S1 A. vanile WT and optic knockout phenotypes in developing wings and body. Ilustrative optix mosaic knockout phenotypes are shown at different stages of development (A) adult, (B) ommochrome stage (when ommochrome pigments first appear). (C) melanin stage (when black melanin pigments first began to appear), and (D) late melanin stage (when black melanin pigments are prevalent across the wing). Arrowheads and dashed lines highlight ilustrative mutant done boundaries. We next aimed to test whether optix regulates wing patterning in more distantly related lineages by performing knockouts in the nymphalines V. cardul (Fig. 2, Fig. 52, and Dataset S1, Tables S1 and S2) and J. conia (Fig. 3, Fig. 53, and Dacase S1, Tables S1 and S2), which diverged from heliconines by 75-80 mya (20, 21). Our results were consistent with those from H. erato and A. vanillae, where optir knockouts in both species showed mutant clones with complete loss of presumptive ommochrome pigments and replacement by melanins (Figs. 2A-E and 3A-C). One interesting exception to this finding was in V. cardul, where the complete ommochrome-to-melanin switch consistently occurred in dorsal wings (Fig. 2A and B), but much of the ventral wing area showed only a loss of ommochrome and little obvious hypermetanization (Fig. 2A, C, and D). Importantly, however, we recovered late-stage pupal wings from V. card that had died before emergence that displayed hypermnelanization of ventral wing surfaces (Fig. 3E). We speculate that this variable strength of ventral wing pattern melanization among individuals may reflect a dosage effect, with the stronger phenotypes representing biallelic optix deletion clones. We have no direct evidence for this, however, given the challenges in rigorously characterizing specific alleles from individual mutant clones (1). We also recovered hypermanic opti knockout pupae in both V. cardul (Fig. S2) and J. coenia (Fig. S3). Taken together, our knockout data from four nymphalids clearly demonstrate that optic plays a conserved role in coordinating the color identities of butterfly wing scales, s, where it operates as an "or" function between ommochrome and melanin identities, but a ut also may be modulated to serve as an "and" function in some contexts, as demonstrated by phenotypes seen in the ventral wings of V. cardu Fig. 2. optic determines wing scale color identity and morphology in V. cardul. (A) optix knockout mutant showing loss of omnochrome pigments (8-0) Left-right asymmetrical comparisons from individual optix mutant butterflies, showing melanization of red pattems (8), loss of color pigmentation without widespread hypermelanization in the ventral forewing (C) and hindwing (D). (E) Severe defects in late-stage pupal wings displaying hypermelanization in sed regions of dorsal and ventral wing surfaces (green and purple arrowheads) compared with mosaic adult mutants in A. (F) opdx knockout showing conversion of pointed wing conjugation scales to normal scales. Fig. 3. optic coordinates pigment color and structural iridescence in J. conia. (A) optix knockout results in loss of red onochrome pigments, r s melanization, and gain of structural iridescence. The left-right comparisons shown are bilaterally asymmetric mosaic phenotypes from single individuals. Wings to the right are additional examples of mosaic mutants with clearly identifiable knockout clones that highlight indescence in both dorsal and ventral wing surfaces. (B-C) Details of mosaic defects in the asymetic mutant shown in A, including strong induced indescence in c in dorsal discal spot (D) and eyespot ring (8), replacement of orange ommochrome with presumptive melanin in ventral discal spot (DI) red patiens (C), and detail of knockout-induced indescence on the ventral hindwing (D). (E) Mosaic knockout clones showing asymmetrical variation in promoted melanin and iridescence induction in dorsal forewings. (F) Wing conjugation scales in WT and optir knockout mutant Fig. 52. V. card WT and optix knockout phenotypes in developing wings and pupae. Mustrative optimes knockout phenotypes are shown at different stages of development (A) adult and pupa. (B) ommochrome stage (when omnochrome pigments first appear). (C) melanin stage (when black melanin pigments first began to appear), and (D) melanin stage (when black melanin pigments are prevalent across the wing). Arrowheads and dashed lines highlight lustrative mutant clone boundaries. The actual wings used for the RNA-seq experiments shown in Fig. 5 are labeled Fig. 52 J.conia WT and optix knockout phenotypes in developing wings and pupae. Mustrative optix mosaic knockout phenotypes are shown at different stages of development (4) whole adults and pupa, (0) details of clone boundaries on adult wings showing iridescent and noniridescent color shifts, and (C) late melanin stage wings (when black melanin pigments are prevalent across the wing). Arrowheads and dashed lines highlight ilustrative mutant clones and boundaries. The actual wings used for RNA-seq experiments in Fig. 5 are labeled "RNA-seq" optix Function Is Required for Determination of Derived Scale Structures. Along with its expression in color patios, in situ optix expression also precisely predicts the location of patches of derived, pointed scales thought to play a role in conjugating forewings and hindwings during fight (5,14). To determine whether optix plays a role in determining the unusual morphology of these scales, we examined optix knockouts for changes in wing scale structure. Indeed, we found that in all four species, optic knockout resulted in transformation of One 1 vanillae (14) scales occurred within obvious knockout clones. Therefore, whether optic plays a functional role in the development of es in transformation of wing conjugation scales to role in switching between discrete complex scale morphologies in buying optix Regulates Iridescence in J. coena The most surprising results from the present study came from our work in 2. conia, where knockout of op induced strong blue indescence in wing scales (Fig. 3A-E and Fig. 5 This induction of structural color occurred in addition to the loss of home pigmentation described above Broad strong descence occumed in knockdal wing surfaces, including in scales that are normally buff or orange in WT bueres, such as the big orange discal spots and eyespot rings (Fig. 3A and d) and the marginal bands of the dorsal hindeling (Fig. 34) descence induction was areas of the wing that are normally heavily melanced in WT indus, such as the black borders of the dial spets regulatory effect. We speculate that these dunes may represent lower dosage effects due to clones being moncadelic for deletion; however, we have not confirmed this hypothesis in sum, our knockout experiments in Jona addition to its other function pigmentation J. ceenia optix Mutants Phenocopy Distantly Related Species. One striking aspect of the J. conie pockout phenotype is the degree to which peoples the arch" tailles. Even singly Rocusing on the nynghald vibe Junonin which includes J. coena phylogenetic analysis suggests Fig 4 Notable examples of fed black and blue phenotypes are seen in such species as Junonia at which are knockouts. As in V card wings and are artholings or panings of putate pigeration genes exqu pignant maturation andor spatially associated with sed and black color sgions in card Using these criteria w ochrome pigmentation and 3 genes associated with melanin pigmentation V porters and one ATP-binding capo fany meber Another strongly down-regulated Juvenile hormone binding pro a gene of unknown function that showed one of the strangest sig expression levels with log-ble change in Pyke FDR yakaca and J. conia ined by local changes in optix expression, although further work is needed to test this hypothes pem density seen in bus candu and showed ively low on hagments per base of transorgt per million mapped pigment pers. Furthermore, our data suggest that there may be differences in the downs of the opt s that we investigated, optikas producing and replaced with black and gray means. Further bucke biochemical, and morphological features, has such a simple an dically alter aange of independent as Compared with her wing body. The behaves the a paidush that can be applied anywhere on a tutely to model and the can be deployed across numerous morphologies to effect disc phenotypic shits through switch- ow a gene underlying adaptive evolution also has a deeply conserved regulatory role in morphological development. In this respect it is investing that opt knockouts phonocopy the di red-black-descent evolutionary color state changes commonly observed in many wing patiem elements, including the whole wing black and blue parchetype that has an on any occasions in buteres. These phenols lead us to hypothesize that evolutionary changes in cox expression may have played a repeated rede in nymphalid wing pate evolution, although additional functional work is needed to rigorously as this hypothes Materials and Methods CRISPR/Cas Genome Editing design and unce Phylogenetic Analysis causing maximum parsimony in the character We first divided merly wings in four segions based border try, and discal spor so called D and D pam) We further classfied the descence vedic 0343234 and depending on the extent of descence occurence in these foun Pupal Wing Isolation and mRNA extraction cadandofwings and hidings were added and stored in The Tybalts were sandbewing and wi the optix knockout effects seen in conia (C) Parsimony reconstruction of indescence in Junoni suggests origins as a fixed phenotype. Species highlighted in red are shown in A. Prevalence of descence analing Global Expression Profiling of Butterfly Wings in Response to optix Knockout. To imate how wing patems are controlled by optix, we used RNA-seq to compare transcript abundance in WT and s are visible in two biological seplicates of both W and strong knockout phenotypes (Figs. 52 and 53 and Dataset 51, Table 5) West examined the expession of op and script ads taled to reveal any paid vansoripes showing lesions at the Cast out site, suggesting expressed genes (DEG) in comparisons between WT and optix knockout wings using cutoff values of a old change of and a false discovery case (FDR) of in card SP ungenes were up-regulated and 243 were dove-regulated inclved in Pane pathway and drophis eye development (1) S1 Table 57 highlights Discussion functional evidence that the widely studied a ot plays a fundamen The ability of a single gene to independ the placement of color anywhere only allo dass of pigments papilodones in papiliorids, prins in pledds, and comes in nymphalids (1) Given th dent origina in butterflies. We propose that opt kaly played a causative role in the origin of wing color in the Nymphalidae, the most speciose and morphologically diverse butterfly family. This gene's simple and deeply conserved regulatory function of replacing melanin with onochrome pigmentation leads to a simple model where co-option of optic into a color patting role could have been the key event sufficient for the deployment of we collected in J. conia Library construction and sequencing were conducted as described previously Analysis of Transcript Expression Data The card come by was downloaded from www.bugome.org and served buldance for conia A-seq analysis sequencing reads from this study and National Cer for Beech using Trinity in scenon The TansDecoder predicted geneset was then searched agai Pan and GO-debuses for gene, domain, and GO annotation, respectively Sequencing data were subrced to quality color and that has also played a nole in color parten evolution in mutiple species (25) The stage of ably and expression profes are able in the GED database accession
Human Heredity: Principles and Issues (MindTap Course List)
11th Edition
ISBN:9781305251052
Author:Michael Cummings
Publisher:Michael Cummings
Chapter13: An Introduction To Genetic Technology
Section: Chapter Questions
Problem 20QP: Analyzing Cloned Sequences A base change (A to T) is the mutational event that created the mutant...
Related questions
Question
(a) What is Figure 1 in the Zheng et al paper showing? Describe it in your own words.
(b) What are some sections of the Zheng paper that are re-arranged or different than you might expect in a primary research article. Is this still primary research?
(c) How did the author use CRISPR to alter the butterfly wing expression? What was the result of this use of CRISPR?

Transcribed Image Text:Significance
The optix gene is well known for its genetic association with wing pattern variation in butterflies; however,
its actual function has never been directly confirmed. Using CRISPR genome editing in multiple butterfly
species, we show that this gene plays a fundamental and deeply conserved role in the butterfly family
Nymphalidae, where it acts as an activator of wing color. We were also surprised to discover that optix
simultaneously controls blue iridescence in some species as well, providing an example of how a single
gene can act as a switch to coordinate between structural and pigmentary coloration.
Abstract
also show that optix simultaneously acts as a switch gene
The optic gene has been implicated in butterfly wing pattern adaptation by genetic association, mapping,
and expression studies. The actual developmental function of this gene has remained unclear, however.
Here we used CRISPR/Cas9 genome editing to show that optix plays a fundamental role in nymphalid
butterfly wing pattern development, where it is
it is required for determination of all chromatic coloration opti
knockouts in four species show of
show complete replacement of color pigments with melanins, with
corresponding changes in pigment-related gene expression, resulting in
g in black and gray butterflies. We
structural iridescence in some
butterflies, demonstrating simple regulatory coordination of structural and pigmentary of
coloration.
Remarkably, these optix knockouts phenocopy the recurring "black and blue" wing pattern archetype that
has arisen on many independent occasions in butterflies. Here we demonstrate a simple genetic basis for
structural coloration, and show that optix plays a deeply conserved role in butterfly wing patter
development.
Sign up for PNAS alerts.
Get alerts for new articles, or get an alert when an article is cited
Butterfly wing patterns provide an important model system for studying the interplay among ecological developmental
and genetic factors in the evolution of complex morphological traits. Dozens of genes have been implicated in wing
pattem development thanks to a combination of comparative expression and, more recently, knockout studies (1-4).
Interestingly, however, mapping and association work has highlighted only a small subset of these genes that seem to
play a
all been genetically a
y associated with local adaptation in multiple
Pedicure gook A, care and doublesex (5-3). These genes are
nadas "adaptive hotspor genes chic logical
and/or r
acrosse
d. based on detailed crossing and expression studies.
these genes behave as complex trait regulators, with different alleles associated with different spatial expression doners
that determine highly varied and complex color patterns, not simply the presence or absence of individual features.
Although there is strong interest in these genes for these reasons, their specific developmental roles and the depth of
conservation of their color patteming functions remain unclear.
Here we present a comparative functional analysis of the optix gene in buterflies. This gene is linked to adaptive
geographic variation of red ommochrome color pattems in the genus Heliconius, although its actual function remained
unconfirmed before the present study (5, 13), optic is al
is also interesting because it is expressed in association with
nonpigmentation wing traits in various species, including morphologically derived wing conjugation scales, suggesting that
it may have multiple regulatory roles in both wing scale coloration and structure (5, 14), in the present work, we used
Case-mediated targeted deletion of optic to test its color pattering function in four species of nymphalid butterflies. Not
only did we confirm deeply conserved roles for optix i
surveyed, but we were surprised to find that in chology in all species
al iridescence in some
butterflies. Importantly, this coordinated regulation of pigmentation and indescence strongly phenocopies wing pattems
seen in other distantly related species, leading us to hypothesize that optix may have played a role in wing pattern
evolution in many different butterfly lineages.
Results
optix Simultaneously Represses Melanins and Promotes Ommochromes.
optix was first identified as a wing pattern gene candidate in Heliconius butterflies, in which mapping, association, and in
situ expression data suggest a role in the determination of red color patterns (5, 14, 15). Subsequent mRNA-seq work
also showed up-regulation of optix in red color patterns of the painted lady butery Vanessa card, raising the possibility
of a more widespread role for this gene in red color pattern specification (16). To functionally confirm the role of optix in
color patterning, we used a Cas-mediated long-deletion mosaic knockout approach (4, 16, 17) in four nymphalid species:
Heliconius erat, Agraulis variae, V. cardul, and Junonia conia (Dataset S1, Tables S1 and S2).
optix knockout in H. erato produced results predicted by previous genetic and in situ hybridization studies. Mosaics
revealed loss of the red color patiems previously shown to be presaged by pupal optix expression, including the color feld
at the base of the forewing (the so-called 'dennis" element) and the hindwing rays (Fig. 1A and B and Dataset S1, Tables
S1 and S2). Not only was red pigmentation lest in knockout clones, but red pigments were replaced by black pigments.
These results show that optix is required for red color pattern specification in H. erato, and acts as a coordinating "or"
switch between ormochrome (orange and red) and melanin (black and gray) pigment types.
Fig. 1.
optix determines wing scale color identity and morphology in H. erato and A. vanilee. (A) optix mosaic knockouts in H
erato result in conversion of red ommochrome color patiems to black melanin. The comparisons shown are left-right
asymmetrical knockout effects from single individual injected buterflies. (B) Detail of mutant clone highlighted in the
mutant in A showing red replaced by black in a proximal red "dennis" pattern of the dorsal forewing. (C-C) optix knockout
mosaics showing transformation of pointed wing conjugation scales to normal wing scales. Each panel in the series
shows successive detail. (D) opdix replaces orange and brown ommochromes in A. vanillae with melonins, resulting in a
black and silver butterfly. Arrows highlight presumptive clone boundaries discussed in the text. () Detail of a knockout
done boundary highlighting the switch between red and black pigmentation in the ventral forewing from D. (F) Ventral
view of black spots in optix knockout mutant showing a phenotype similar to WT. (G and G) Wing conjugation scales in
WT (G) and optix knockout mutant (G) demonstrating a role for optic in determining A. vanilla scale morphology
To test whether optix has a role in color paterning in a more basal heliconine butterfly, we generated knockouts in the gulf
fritillary A. vanilla (Fig. 1D-F, Fig. S1, and Dataset 51, Tables S1 and S2). Previous in situ hybridization work in A
vanilla suggested that optic is not expressed in association with ommochrome patems during early pupal development.
leading to the hypothesis that the gene might not play a major color patterning role in this species (14). Thus, we were
surprised to find that optix knockout resulted in a complete transformation of ommochrome scales to black melanin scales,
producing a very unusual and dramatic phenotype of a completely black and silver butterfly (Fig. 10). We also observed a
handful of orange or brown scales that changed to silver patches (Fig. 10, ventral forewing.
1. green arrows), although we
cannot confidently conclude that these are cell-autonomous knockout effects s
3 since it has been shown that silver scales
can be induced through long-range signaling (18, 19), in this case potentially from neighboring knockout clones. The with
type (WT) black spots and marginal bands in the ventral forewing were unaffected in knockouts and remained a darker
color relative to the neighboring mutant melanic scales (Fig. 1), optix knockout also resulted in melanic
hyperpigmentation in adult bodies (Fig. S14). Thus, our results in A. vanillae are consistent with those in Herato in
supporting a role for optix as a switch-like regulator that toggles between ormochrome and melanin pattems.
Fig. S1
A. vanile WT and optic knockout phenotypes in developing wings and body. Ilustrative optix mosaic knockout
phenotypes are shown at different stages of development (A) adult, (B) ommochrome stage (when ommochrome
pigments first appear). (C) melanin stage (when black melanin pigments first began to appear), and (D) late melanin stage
(when black melanin pigments are prevalent across the wing). Arrowheads and dashed lines highlight ilustrative mutant
done boundaries.
We next aimed to test whether optix regulates wing patterning in more distantly related lineages by performing knockouts
in the nymphalines V. cardul (Fig. 2, Fig. 52, and Dataset S1, Tables S1 and S2) and J. conia (Fig. 3, Fig. 53, and
Dacase S1, Tables S1 and S2), which diverged from heliconines by 75-80 mya (20, 21). Our results were consistent
with those from H. erato and A. vanillae, where optir knockouts in both species showed mutant clones with complete loss
of presumptive ommochrome pigments and replacement by melanins (Figs. 2A-E and 3A-C). One interesting exception
to this finding was in V. cardul, where the complete ommochrome-to-melanin switch consistently occurred in dorsal wings
(Fig. 2A and B), but much of the ventral wing area showed only a loss of ommochrome and little obvious
hypermetanization (Fig. 2A, C, and D). Importantly, however, we recovered late-stage pupal wings from V. card that had
died before emergence that displayed hypermnelanization of ventral wing surfaces (Fig. 3E). We speculate that this
variable strength of ventral wing pattern melanization among individuals may reflect a dosage effect, with the stronger
phenotypes representing biallelic optix deletion clones. We have no direct evidence for this, however, given the challenges
in rigorously characterizing specific alleles from individual mutant clones (1). We also recovered hypermanic opti
knockout pupae in both V. cardul (Fig. S2) and J. coenia (Fig. S3). Taken together, our knockout data from four
nymphalids clearly demonstrate that optic plays a conserved role in coordinating the color identities of butterfly wing
scales,
s, where it operates as an "or" function between ommochrome and melanin identities, but a
ut also may be modulated to
serve as an "and" function in some contexts, as demonstrated by phenotypes seen in the ventral wings of V. cardu
Fig. 2.
optic determines wing scale color identity and morphology in V. cardul. (A) optix knockout mutant showing loss of
omnochrome pigments (8-0) Left-right asymmetrical comparisons from individual optix mutant butterflies, showing
melanization of red pattems (8), loss of color pigmentation without widespread hypermelanization in the ventral forewing
(C) and hindwing (D). (E) Severe defects in late-stage pupal wings displaying hypermelanization in sed regions of dorsal
and ventral wing surfaces (green and purple arrowheads) compared with mosaic adult mutants in A. (F) opdx knockout
showing conversion of pointed wing conjugation scales to normal scales.
Fig. 3.
optic coordinates pigment color and structural iridescence in J. conia. (A) optix knockout results in loss of red
onochrome pigments, r
s melanization, and gain of structural iridescence. The left-right comparisons shown are bilaterally
asymmetric mosaic phenotypes from single individuals. Wings to the right are additional examples of mosaic mutants with
clearly identifiable knockout clones that highlight indescence in both dorsal and ventral wing surfaces. (B-C) Details of
mosaic defects in the asymetic mutant shown in A, including strong induced indescence in c
in dorsal discal spot (D) and
eyespot ring (8), replacement of orange ommochrome with presumptive melanin in ventral discal spot (DI) red patiens
(C), and detail of knockout-induced indescence on the ventral hindwing (D). (E) Mosaic knockout clones showing
asymmetrical variation in promoted melanin and iridescence induction in dorsal forewings. (F) Wing conjugation scales in
WT and optir knockout mutant
Fig. 52.
V. card WT and optix knockout phenotypes in developing wings and pupae. Mustrative optimes knockout
phenotypes are shown at different stages of development (A) adult and pupa. (B) ommochrome stage (when
omnochrome pigments first appear). (C) melanin stage (when black melanin pigments first began to appear), and (D)
melanin stage (when black melanin pigments are prevalent across the wing). Arrowheads and dashed lines highlight
lustrative mutant clone boundaries. The actual wings used for the RNA-seq experiments shown in Fig. 5 are labeled
Fig. 52
J.conia WT and optix knockout phenotypes in developing wings and pupae. Mustrative optix mosaic knockout
phenotypes are shown at different stages of development (4) whole adults and pupa, (0) details of clone boundaries on
adult wings showing iridescent and noniridescent color shifts, and (C) late melanin stage wings (when black melanin
pigments are prevalent across the wing). Arrowheads and dashed lines highlight ilustrative mutant clones and
boundaries. The actual wings used for RNA-seq experiments in Fig. 5 are labeled "RNA-seq"
optix Function Is Required for Determination of Derived Scale Structures.
Along with its expression in color patios, in situ optix expression also precisely predicts the location of patches of
derived, pointed scales thought to play a role in conjugating forewings and hindwings during fight (5,14). To determine
whether optix plays a role in determining the unusual morphology of these scales, we examined optix knockouts for
changes in wing scale structure. Indeed, we found that in all four species, optic knockout resulted in transformation of
One
1 vanillae (14)
scales occurred within obvious knockout clones. Therefore, whether optic plays a functional role in the development of
es in transformation of wing conjugation scales to
role in switching between discrete complex scale morphologies in buying
optix Regulates Iridescence in J. coena
The most surprising results from the present study came from our work in 2. conia, where knockout of op induced
strong blue indescence in wing scales (Fig. 3A-E and Fig. 5 This induction of structural color occurred in addition to
the loss of home pigmentation described above Broad strong descence occumed in knockdal
wing surfaces, including in scales that are normally buff or orange in WT bueres, such as the big orange discal spots
and eyespot rings (Fig. 3A and d) and the marginal bands of the dorsal hindeling (Fig. 34) descence induction was
areas of the wing that are normally heavily melanced in WT indus, such as the black borders of the dial spets
regulatory effect. We speculate that these dunes may represent lower dosage effects due to clones being
moncadelic for deletion; however, we have not confirmed this hypothesis in sum, our knockout experiments in Jona
addition to its other function pigmentation
J. ceenia optix Mutants Phenocopy Distantly Related Species.
One striking aspect of the J. conie pockout phenotype is the degree to which peoples the arch"
tailles. Even singly Rocusing on the nynghald vibe Junonin which includes J. coena phylogenetic analysis suggests
Fig 4 Notable examples of fed black and blue phenotypes are seen in such species as Junonia at which are
knockouts. As in V card
wings and are artholings or panings of putate pigeration genes exqu
pignant maturation andor spatially associated with sed and black color sgions in card Using these criteria w
ochrome pigmentation and 3 genes associated with melanin pigmentation V
porters and one ATP-binding capo fany meber Another strongly down-regulated
Juvenile hormone binding pro a gene of unknown function that showed one of the strangest sig
expression levels with log-ble change in Pyke FDR yakaca and J. conia
ined by local changes in optix expression, although further work is needed to test this hypothes
pem density seen in bus
candu and showed ively low on hagments per base of transorgt per million mapped
pigment pers. Furthermore, our data suggest that there may be differences in the downs of the opt
s that we investigated, optikas producing
and replaced with black and gray means. Further bucke
biochemical, and morphological features, has such a simple an
dically alter aange of independent as Compared with her wing
body. The behaves the a paidush that can be applied anywhere on a tutely to model and the
can be deployed across numerous morphologies to effect disc phenotypic shits through switch-
ow a gene underlying adaptive evolution also has a deeply conserved
regulatory role in morphological development. In this respect it is investing that opt knockouts phonocopy the di
red-black-descent evolutionary color state changes commonly observed in many wing patiem elements, including
the whole wing black and blue parchetype that has an on any occasions in buteres. These phenols
lead us to hypothesize that evolutionary changes in cox expression may have played a repeated rede in nymphalid wing
pate evolution, although additional functional work is needed to rigorously as this hypothes
Materials and Methods
CRISPR/Cas Genome Editing
design and unce
Phylogenetic Analysis
causing maximum parsimony in the
character We first divided merly wings in four segions based
border try, and discal spor so called D and D pam) We further classfied the descence
vedic 0343234 and depending on the extent of descence occurence in these foun
Pupal Wing Isolation and mRNA extraction
cadandofwings and hidings were added and stored in The
Tybalts were sandbewing and wi
the optix knockout effects seen in conia (C) Parsimony reconstruction of indescence in Junoni suggests
origins as a fixed phenotype. Species highlighted in red are shown in A. Prevalence of descence analing
Global Expression Profiling of Butterfly Wings in Response to optix Knockout.
To imate how wing patems are controlled by optix, we used RNA-seq to compare transcript abundance in WT and
s are visible in two biological seplicates of both W and strong
knockout phenotypes (Figs. 52 and 53 and Dataset 51, Table 5) West examined the expession of op
and
script ads taled to reveal any paid vansoripes showing lesions at the Cast out site, suggesting
expressed genes (DEG) in comparisons between WT and optix knockout wings using cutoff values of a old change of
and a false discovery case (FDR) of in card SP ungenes were up-regulated and 243 were dove-regulated
inclved in Pane pathway and drophis eye development (1) S1 Table 57 highlights
Discussion
functional evidence that the widely studied a
ot plays a fundamen
The ability of a single gene to independ
the placement of color anywhere only allo
dass of pigments papilodones in papiliorids, prins in pledds, and comes in nymphalids (1) Given th
dent origina in butterflies. We propose that opt kaly played a causative role in the origin of
wing color in the Nymphalidae, the most speciose and morphologically diverse butterfly family. This gene's simple and
deeply conserved regulatory function of replacing melanin with onochrome pigmentation leads to a simple model where
co-option of optic into a color patting role could have been the key event sufficient for the deployment of
we collected in J. conia Library construction and sequencing were conducted as described previously
Analysis of Transcript Expression Data
The card come by was downloaded from www.bugome.org and served
buldance for conia A-seq analysis sequencing reads from this study and National Cer for Beech
using Trinity in scenon The TansDecoder predicted geneset was then searched agai
Pan and GO-debuses for gene, domain, and GO annotation, respectively Sequencing data were subrced to quality
color and that has also played a nole in color parten evolution in mutiple species (25) The stage of
ably and expression profes are able in the GED database accession
AI-Generated Solution
Unlock instant AI solutions
Tap the button
to generate a solution
Recommended textbooks for you
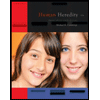
Human Heredity: Principles and Issues (MindTap Co…
Biology
ISBN:
9781305251052
Author:
Michael Cummings
Publisher:
Cengage Learning
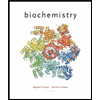
Biochemistry
Biochemistry
ISBN:
9781305577206
Author:
Reginald H. Garrett, Charles M. Grisham
Publisher:
Cengage Learning
Case Studies In Health Information Management
Biology
ISBN:
9781337676908
Author:
SCHNERING
Publisher:
Cengage
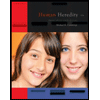
Human Heredity: Principles and Issues (MindTap Co…
Biology
ISBN:
9781305251052
Author:
Michael Cummings
Publisher:
Cengage Learning
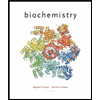
Biochemistry
Biochemistry
ISBN:
9781305577206
Author:
Reginald H. Garrett, Charles M. Grisham
Publisher:
Cengage Learning
Case Studies In Health Information Management
Biology
ISBN:
9781337676908
Author:
SCHNERING
Publisher:
Cengage