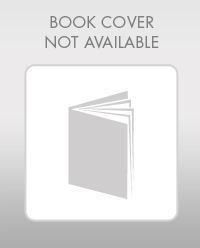
In response to the increasing challenges in water service provision, there has been a significant shift advocated in Urban Water Management (UWM) globally, recognized as a critical necessity for cities. Particularly addressing the urban drainage aspect, a myriad of analogous urban planning and design methodologies for decentralized, environmentally friendly systems have emerged worldwide (Fletcher et al., 2014). These methodologies include Sustainable Urban Drainage Systems (SUDS) (Woods-Ballard et al., 2007), Low Impact Development (LID) (US EPA, 2000), Green Infrastructure (GI) (Benedict and McMahon, 2012), Best Management Practice (BMP) (US EPA, 2011), Water Sensitive Urban Design (WSUD) (Wong, 2006), and Sponge City (Liu et al., 2022). Despite variances in scope and context among these concepts, they share a fundamental philosophy: rather than disregarding the natural hydrological cycle, they depend on the "activation of natural processes" (Fryd et al., 2012).
In recent years, the intersecting role of greener innovations in promoting financial inclusion has gained attention (Brahmi et al, 2023). Regulatory support is essential to harness the full potential of greener innovations for achieving environmental, economic, and social sustainability.In this context, Aldieri et al. (2021) propose that the government could establish dedicated funds to incentivize companies to enhance their innovative activities within the production process (Aldieri et al.,2021). Additionally, Brahmi and Alderi (2023) have underscored the importance of governance
This approach is implemented through both structural (green infrastructure systems such as rain gardens and wetlands) and non-structural measures (policies aimed at enhancing water use efficiency) (Beecham, 2003; Butler and Memon, 2006; Taylor and Wong, 2002; Stevens et al., 2012). Wang and Brown's (2009) advocacy for sustainable urban water management principles emphasizes the significance of having access to a variety of water sources, providing ecological services, and utilizing socio-political capital in order to achieve sustainability.
Water Sensitive Cities (WSC) has emerged as a promising approach to urban water management, offering multifaceted benefits such as water conservation, stormwater quality improvement, flood control, landscape amenity, and enhanced living environments (Sharma et al., 2016; Ashley et al., 2004; Fryd et al., 2012; Martin et al., 2007; Wong et al., 2013; Wong and Brown, 2009; Woods-Ballard et al., 2007). While WSC systems exhibit significant potential, their mainstream uptake faces several knowledge gaps across technical, economic, social, and institutional domains (Sharma et al., 2016). To fill up these gaps, Rogers (2020) introduced the Water Sensitive Cities Index, a diagnostic tool for assessing water sensitivity and guiding management activities. The index encompasses various goals, including governance, community capital, equity of services, resource efficiency, ecological health, urban spaces, and adaptive infrastructure. In essence, the literature demonstrates the evolution of water-sensitive cities and its integral role in sustainable urban water management. From technical innovations to adaptive planning frameworks, a holistic approach is essential to realize the vision of water-sensitive cities and tackle complex urban challenges.
Methodology:
2.1 Data Sources and Selection Process
This study leveraged data sourced from the Web of Science (WoS) database, selected for its extensive coverage of peer-reviewed articles across diverse research fields. The comprehensive details provided by WoS, encompassing information on authors, institutions, countries, journals, and citations, make it a fitting source for this research (Yang et al., 2021). On December 10th, 2023, the data collection process involved a topic search that integrated the title, abstract, and keywords. The search terms employed included "water-sensitive city," "water-sensitive urban planning," and "water-sensitive urban design." Following a meticulous review of publication titles and the selection of pertinent articles, a total of 707 articles were incorporated into the database.

Step by stepSolved in 2 steps with 4 images

- The 60-year record shows that the annual maximum discharges for a river have a mean of 30,000 cfs, a standard deviation of 28,000 cfs, and a skewness coefficient of 3.15. If the annual maximum discharge can be described by the Extreme-value Type I distribution, what is the probability that the annual maximum discharge exceeds 100,000 cfs at least once in next 10 years? (Note: The sample size is 60. Conduct interpolation using exceedance probabilities or cumulative probabilities.)arrow_forwardHow would you go about deriving a UH from a catchment for which data (catchment area, rainfall and discharge values) are available? Derive a hyetograph from the incident rainfall (losses do not need to be considered to derive a UH); Determine the UH using the formula "Ui = Qi/P" (for a single interval hyetograph) or using "deconvolution" (for a multiple interval hyetograph). Estimate direct runoff hydrograph by subtracting baseflow from observed stream flow data; Estimate the total runoff volume and the direct runoff depth over the catchment; Determine the UH ordinates using the formula "Unit hydrograph = Direct runoff hydrograph x 1/Direct runoff depth"; Estimate catchment losses and subtract these from the incident precipitation to obtain excess rainfall; the duration of the excess rainfall is used as the duration of the UH. Determine the precipitation volume for each time interval; Use UH superposition and proportionality principles to derive a direct unit hydrograph; Estimate…arrow_forwardDifferent steps of watershed modeling are provided in the options below. Select the option that is related to the last steps of the watershed modeling. O Evaluation and refinement of study objectives Sensitivity analysis, and usefulness evaluation Model selection, and input data gathering O Method selection, and model calibrationarrow_forward
- Structural Analysis (10th Edition)Civil EngineeringISBN:9780134610672Author:Russell C. HibbelerPublisher:PEARSONPrinciples of Foundation Engineering (MindTap Cou...Civil EngineeringISBN:9781337705028Author:Braja M. Das, Nagaratnam SivakuganPublisher:Cengage Learning
- Fundamentals of Structural AnalysisCivil EngineeringISBN:9780073398006Author:Kenneth M. Leet Emeritus, Chia-Ming Uang, Joel LanningPublisher:McGraw-Hill EducationTraffic and Highway EngineeringCivil EngineeringISBN:9781305156241Author:Garber, Nicholas J.Publisher:Cengage Learning
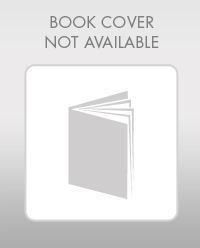
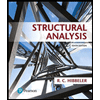
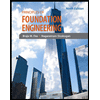
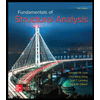
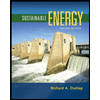
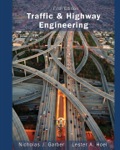